Amy Sprague
June 27, 2024
A&A researchers look to stingrays for how to develop efficient, quiet and sustainable underwater vehicles.
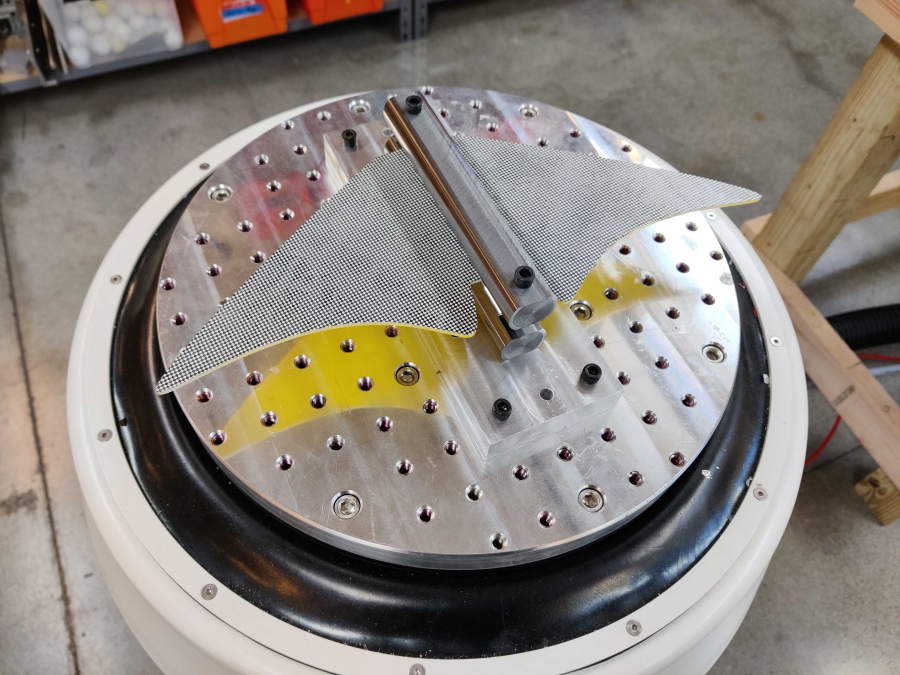
Fin model of Mobula birostris affixed to the Illimited Lab’s Data Physics 400V Shaker Table for testing using a custom edge mount.
By studying the unique swimming abilities of stingrays and other batoids, A&A researchers hope to unlock the secrets of efficient undulatory propulsion that could lead to a new generation of quiet and sustainable underwater vehicles.
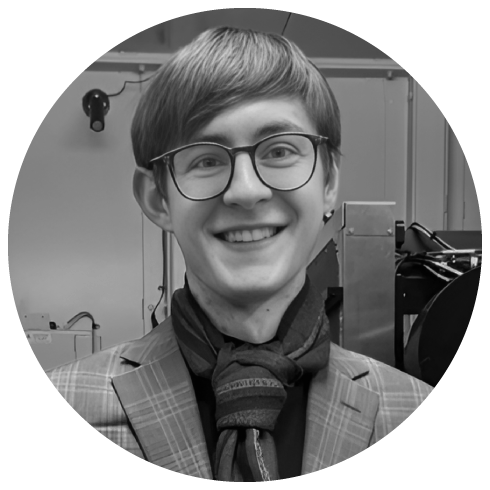
“There's an extraordinary diversity of body shapes, skeletal structures and swimming modes across the different batoid species. By systematically analyzing and comparing them, we can gain insights to apply to bio-inspired robotic designs adapted for different scenarios and environments.”
"Stingrays are fast, efficient and maneuverable swimmers that propel themselves by undulating their pectoral fins," explains John Michael Racy, a mechanical engineering undergraduate and researcher in A&A's Illimited Lab. "We want to understand the structural dynamics they use to achieve this elegant mode of propulsion."
Racy and his collaborators, including Ph.D. candidate Bart Boom, and faculty advisers Ed Habtour and Adam Summers, are taking an interdisciplinary approach, combining biological studies of batoid anatomy with physics-based modeling and experimental testing. The goal is to translate the rays' unique structural and dynamic characteristics into practical engineering designs.
Current underwater unmanned vehicle designs utilizing undulatory "fin" propulsion tend to apply a brute force actuation approach, which requires complex arrays of bulky and heavy actuators and control systems to induce the wavelike motions. In contrast, batoid rays seem to achieve similar motion more simply, by recycling the energy from ocean waves and internal dynamics. The team believes that energy recycling is accomplished through a sophisticated network of specialized musculoskeletal elements designed to intentionally synchronize their dynamics to harness the energy as much as possible.
"Our hypothesis is that batoids have evolved self-actuating 'fin-wings' where the smart heterogeneous stiffness distribution induces chordwise traveling waves from simple base oscillations," says Racy. "We first achieved that effect in simple experimental and finite element models last year while working with the Washington NASA Space Grant Consortium. If we can further develop the concept, it could allow dramatically simpler actuation and control for robotic undulatory propulsion."
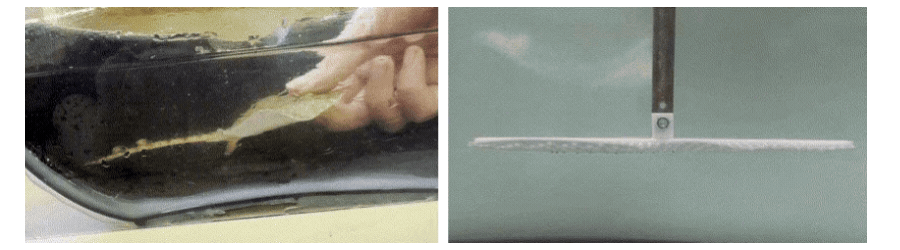
Left: A skate’s fins undulate without conscious control. Right: A highly simplified, 3D-printed representation of a batoid skeleton undulates in the Friday Harbor Labs Flume. Note the similarity in undulation shape to that of the skate.
The team starts by using mathematical modeling techniques like Bezier curves to capture the complex curvatures and stiffness variations of the batoid fins and musculoskeletal elements. From there, they fabricate simplified physical prototypes using laser-cut bonded plates to experimentally test out the predicted dynamic behavior.
“Using high-speed videography of our prototypes under simulated ocean wave excitation, we can then analyze the relationships between the structural patterns and their dynamics”
“Expanding on this method, we can create macro-scale structural dynamics models that are essentially 'skeletons' exhibiting key design characteristics of their musculoskeletal elements. This summer, we are collaborating with the KAUST Splash Lab, led by Professor Tadd Truscott, to design and test these at the Comparative Vertebrate Biomechanics Lab at the Friday Harbor Laboratories."

Illimited Lab Ph.D. candidate Bart Boom records a random excitation trial of a fin model at 8000 frames per second.
According to the Professor Habtour, this project's interdisciplinary melding of biology, dynamics, and engineering holds tremendous potential: "By uncovering the core mechanisms behind the batoids' extraordinary swimming talents, John Michael and his team aren't just increasing our scientific understanding of morphological dynamics - their insights could catalyze transformation in underwater vehicle design."
The work has both civilian and military applications, from more efficient autonomous survey or inspection vehicles, to quieter boats or wave driven ships, to entirely new classes of high-performance underwater vehicles. Racy acknowledges inspiration from groups like DARPA, who have funded related robotics initiatives like the recently unveiled Manta Ray UUV program inspired by batoid forms.
"Stingrays and their cousins have had millions of years to both diversify and optimize their natural propulsion mechanisms," says Racy. "If we can successfully decode it, we can engineer underwater propulsion that is faster, more maneuverable, quieter, more efficient, and easier to control than anything currently possible."
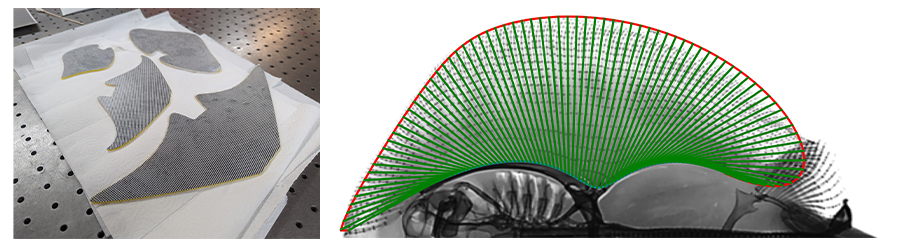
Left: Laser cut acrylic physical models generated from the fin shapes of Mobula birostris (front), Aetomylaeus nichofii (center), Gymnura crebipunctata (back right), and Dasyatis acutirostra (back left). Checkerboard pattern enables optical tracking for dynamic analysis. Right: Overlay of the generated paths between fin base and edge to simulate the architecture of the fin of Urobatis Maculatus.